Molecular interactions: Dance of Entropy and Enthalpy
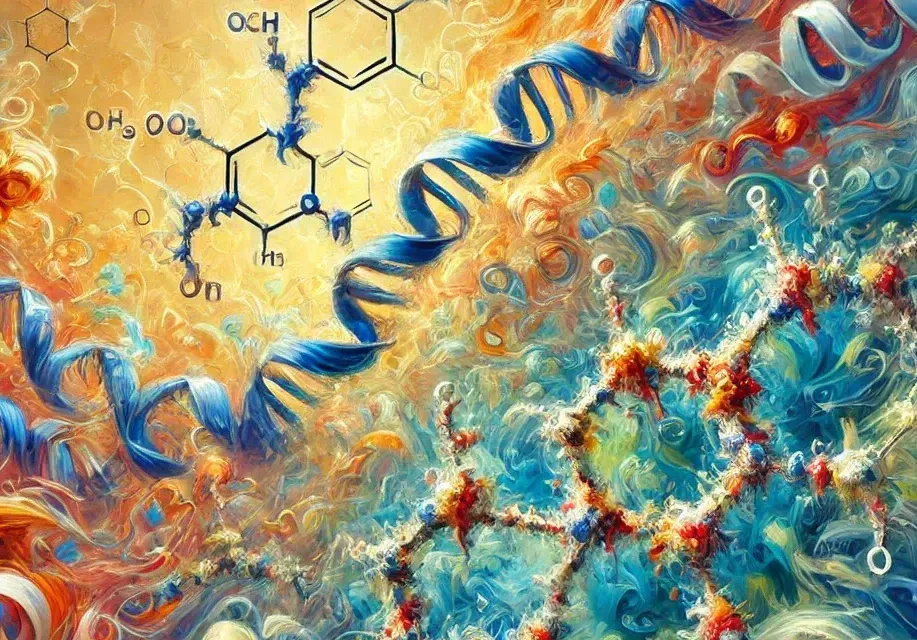
In structure-based drug design, it's easy to be misled by the static image of a crystal structure. When aiming to improve the binding between a protein and a synthetic molecule, our natural instinct is often to increase as many hydrogen-bond interactions as possible by adding additional H-bond donors and acceptors. However, this approach doesn't always yield the desired results. The binding between a protein and a ligand is influenced by numerous factors, including the solvation of both partners, various non-bonded interactions, and the degree of freedom of movement of both the protein and the ligand.
If you've ever felt lost when terms like entropy and enthalpy come up during meetings with experienced drug discovery scientists, you're in the right place. In this post, we'll demystify the basics of molecular interactions and simplify the concepts of enthalpy and entropy in binding.
Binding Free Energy: The Big Picture
Binding free energy (ΔG) is the difference in free energy between the bound state (where the ligand is bound to the protein) and the unbound state (where the ligand and protein are separate). For a binding event to be favourable, this energy difference must be negative. The binding free energy is the sum of two main components: enthalpy term (ΔH) and entropy term(-TΔS). These two forces often work in tandem, and sometimes they oppose each other, leading to a delicate balance that determines the stability of the molecular interaction.
Enthalpy: The Energy of Interaction
Enthalpy represents the energy that is absorbed or released during the binding process. When a ligand binds to a protein, for example, certain interactions, like hydrogen bonds or van der Waals or electrostatic interaction, can form between them. If these interactions are strong, the system releases energy, making the binding process enthalpically favourable. This release of energy usually makes the binding event more stable, driving the two molecules closer together.
However, enthalpy isn’t the whole story. Sometimes, even if the binding is enthalpically favourable (i.e., releasing energy), the system might resist this change. That’s where entropy comes in.
Entropy: The Measure of Disorder
Entropy measures the disorder in a system. In binding, it often relates to the loss of freedom that molecules experience when they bind. Before binding, both the protein and the ligand can move freely; after binding, their movements are restricted. This restriction leads to a decrease in entropy, which can negatively impact binding affinity. Interestingly, proteins sometimes counterbalance this unfavourable entropy decrease within the binding pocket by redistributing dynamics to other parts of the protein.
Sometimes the binding process can actually increase the entropy of the system. This can happen in cases where binding leads to the release of water molecules from a binding site, allowing them to return to the more disordered bulk solution. This entropy gain can counterbalance the entropy loss associated with the restricted movement of the bound molecules.
Water's Influence on Binding
Water molecules play a crucial role in binding interactions. Before a ligand can bind to a protein, both must get rid of some of the water molecules that surround them—a process known as desolvation. This desolvation can be energetically costly, especially if the water molecules are tightly bound to the protein or ligand. If the cost of desolvation is too high, it can prevent binding altogether, even if the ligand appears to fit perfectly in the binding site.
It’s essential to consider this when you're tempted to add basic or acidic groups to your molecule to interact with basic or acidic amino acid side chains, especially on the protein surface. There is a significant desolvation penalty, which is why forming salt bridges doesn’t always enhance potency.
In addition to desolvation, the hydrophobic effect is another key player in binding interactions. This effect describes the tendency of nonpolar molecules or groups to cluster together in water, minimizing their exposure to the solvent. The hydrophobic effect is typically seen as entropy-driven, with water molecules becoming more disordered when hydrophobic groups come together. However, studies using ITC (Isothermal Titration Calorimetry) suggest that the hydrophobic effect can also have significant enthalpic component. Nevertheless, the key takeaway is that water molecules are important and play a major role in binding free energies.
Several computational tools help predict whether to displace water from the binding site, with WaterMap from Schrödinger being one of the most widely used methods in the industry.
Reducing rotational freedom
In structure-based drug design, it’s common practice to reduce the rotational freedom of a ligand by matching its conformation to the bound state within the protein. This restriction is often achieved by adding intramolecular hydrogen bonds, adding steric bulk, or forming macrocycles. Recently, I was asked whether this approach—restricting a ligand's conformation to its biologically active form—might reduce entropy and thereby decrease binding affinity.
At first glance, the idea seems counterintuitive—wouldn’t reducing conformational freedom lower entropy? Not exactly. It’s important to consider the ligand’s rotational freedom in both its bound and unbound states. If the ligand is already somewhat pre-organized or rigid in its unbound state, the conformational change upon binding is smaller, resulting in a less significant loss of entropy. Consequently, the energy required to achieve the binding conformation is reduced, potentially leading to higher binding affinity.
Wrapping Up
By now, you should have a clearer understanding of the basics of molecular interactions, particularly the concepts of enthalpy and entropy. If you're eager to dive deeper, I highly recommend this excellent review. Improving molecular interactions is all about balancing the energy of interactions (enthalpy) with the flexibility and disorder of the entire complex, including solvent (entropy). By carefully considering these forces and how they work together, you can design drugs that not only fit their targets but do so in a way that’s energetically favourable and highly effective.
If you've enjoyed this article and other posts on MoleculeInsight, please consider subscribing and following our LinkedIn and Twitter pages. Your support is invaluable in helping us continue to write on drug discovery topics.